There’s unexpected movement in the world of cell biology — specifically, with the energy factories known as mitochondria.
Ever since they were discovered in the mid-nineteenth century, mitochondria have been known as organelles that reside inside cells. But that textbook picture now seems to be wrong. An explosion of research is challenging mitochondria’s long-standing image as exclusively cellular organelles. “They may be a multicellular organelle,” says Jonathan Brestoff, an immunologist who studies metabolism at Washington University in St. Louis, Missouri. In other words, the supposedly static energy factories now seem to be expert travellers, skipping from one cell to another on demand.
Cancer cells ‘poison’ the immune system with tainted mitochondria
This ‘mitochondrial transfer’ has been observed in a wide variety of cells and in organisms as diverse as yeast, molluscs and rodents. “It’s really exciting to see,” says Jeffrey Spees, a stem-cell biologist at the University of Vermont in Burlington.
It’s not yet clear why mitochondria are so mobile. Some studies have hinted that cells donate their mitochondria to their neighbours during times of need. In cellular emergencies, newly arrived mitochondria might kick-start tissue repair, fire up the immune system or rescue distressed cells from death. Other research suggests that mitochondrial transfer can be a lethal weapon that cancer cells deploy to gain an advantage.
But what this means for human health is still a mystery. Researchers haven’t yet captured the process inside the human body and so don’t know for sure if it happens in people, says Daniel Davis, an immunologist at Imperial College London. “We don’t have the technology yet to witness this happening,” he says.
That fact hasn’t stopped researchers from exploring how to leverage mitochondrial transfer to treat a variety of diseases, including cancer and stroke.
A borrowed bacterium
Over the past three decades, research has revealed that mitochondria are much more than cellular powerhouses that turn nutrients from food into energy. They’re key players in multiple processes in the body: they guide the chatter that keeps cells functioning and contribute to the immune response against harmful invaders. They’re also unexpectedly diverse. Last year, researchers found that mitochondria divide themselves into two distinct forms to help cells survive nutrient starvation1. Another study, published in March, modelled the density and type of mitochondria throughout the human brain2.
All mitochondria — in whatever organism, in whatever part of the body — are thought to have sprung from the same ancient bacterium. Around 1.5 billion years ago, this drifting bacterium was swallowed up by the microbe that eventually gave rise to eukaryotes — the large group of organisms, including us, whose cells have an enclosed nucleus.
Scientists make precise gene edits to mitochondrial DNA for first time
After several evolutionary twists and turns, this borrowed bacterium became the organelle that drives metabolism. Mitochondria’s microbial origins probably help to explain why they are more dynamic than they first appear, says Kazuhide Hayakawa, a neuroscientist at Massachusetts General Hospital in Boston, who studies how mitochondrial transfer could help to treat stroke. “It is possible that mitochondria retain the ancient ability to spread from cell to cell, like bacteria,” he says.
In 2006, Spees and his colleagues captured the first glimpse of mitochondria skipping from one cell to another3. The team had been trying to understand a perplexing behaviour of stem cells in laboratory dishes. These cells seemed to be sharing some kind of physical information that told them how to differentiate, and mitochondria were thought to be involved.
To investigate mitochondria’s role, the researchers cultured human lung cancer cells, which lacked the organelles, with stem cells sourced from bone marrow. With the stem cells’ mitochondria tagged with fluorescent proteins, the team shot a time-lapse video of what happened next.
The grainy black and white footage showed the stem cells shooting out their mitochondria, which were then taken up by the defective lung cells. After the donation, the lung cells quickly recovered their ability to divide and transform glucose into energy. “Watching it was like a miracle,” says Spees.
Since then, researchers have observed mitochondria zipping between several types of cell — lung, heart, brain, fat, bone and more. Sometimes the mitochondria are travelling down ephemeral highways known as tunnelling nanotubes that form between cells and transport other cellular cargo. In other cases, the mitochondria take their journey in bubble-shaped vesicles or float freely in blood4 (see ‘Three ways to go’).
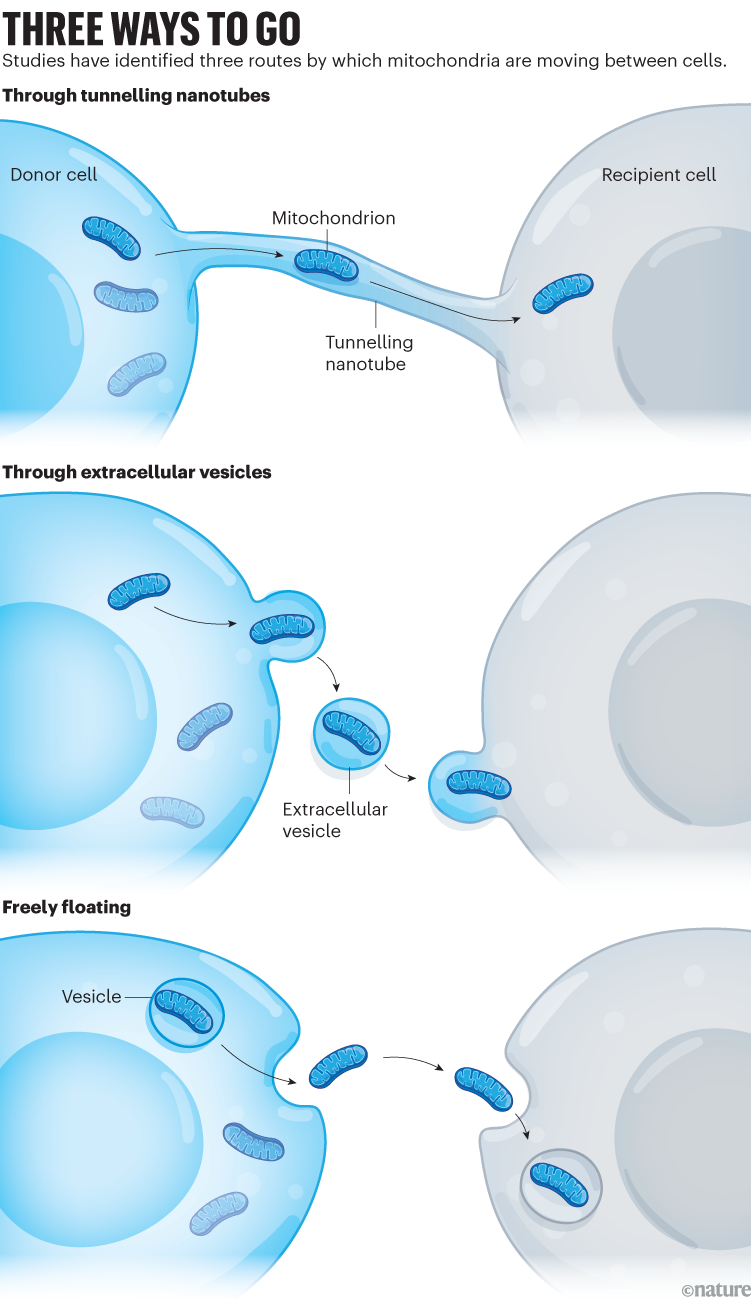
How mitochondria are getting around is largely settled, but what’s less clear is why. Researchers are learning that the process is often a form of cellular damage control, says Clair Crewe, a cell biologist at Washington University.
Some studies suggest, for example, that mitochondrial transfer might help cells to weather neurological storms. In 2016, Hayakawa and his colleagues found that in mice that have had a stroke, support cells called astrocytes deliver their mitochondria to faltering neurons. With this mitochondrial boost, neurons grew branches and restarted their metabolic processes, which improved their chances of survival. When the researchers inhibited mitochondrial transfer, fewer neurons survived, suggesting the donated organelles were key to the cells’ recovery5. But which part of the mitochondria’s structure or function protected the cells remains unknown, says Hayakawa.
Lung cells might also benefit from a mitochondrial boost during a crisis, says Jahar Bhattacharya at Columbia University in New York City, who specializes in a severe inflammatory condition known as acute lung injury. He and his colleagues have found that in mice with this inflammation, stromal cells — which make up connective tissues that support organs — transfer their mitochondria to lung cells6. Cells with loaned organelles had higher concentrations of the cellular fuel ATP, which ended up being distributed to nearby cells that did not receive new mitochondria.
These diseased lungs showed more signs of recovery than did diseased lungs that didn’t receive outside mitochondria. Bhattacharya was amazed when he and his team witnessed mitochondrial transfer in action. “I don’t think we slept for the next few nights, it was so exciting,” he says.
Other research hints that transferred mitochondria might supercharge wound healing. In 2021, Anne-Marie Rodriguez, a cell biologist at Sorbonne University in Paris, and her colleagues found that platelets isolated from human blood shuttled their mitochondria to stem cells when researchers put the two cell types into a dish together. After taking up the mitochondria, the stem cells released molecules that play a part in forming new blood vessels. When the cells were placed onto skin wounds in mice, those injuries healed faster than did injuries in rodents that had received either stem cells or platelets alone7.
Researchers suspect that cells with dysfunctional mitochondria might even have ways to request healthy mitochondria from their neighbours, although the exact mechanisms underlying this process remain murky. “We’re only beginning to understand the signalling that’s involved,” says Crewe.
Everyday operations
Beyond its role in recovery, researchers want to know whether mitochondrial transfer is an essential part of everyday biology. Initial evidence suggests it might help to maintain healthy tissues. Last year, Minghao Zheng, a regenerative biologist at the University of Western Australia in Perth, and his colleagues discovered that some types of astrocyte donate their mitochondria to cells that line blood vessels in the mouse brain8. When the researchers disrupted this process, the blood–brain barrier became leaky, suggesting that mitochondrial transfer helps to maintain this protective membrane shield. Zheng and his team had already reported that mitochondrial transfer in the bones of mice can accelerate the formation of new blood vessels9.
In healthy mice, Brestoff and his colleagues reported, white fat cells transfer their mitochondria to macrophages — white blood cells that hoover up cellular debris. The number of shuttled organelles was reduced in obese mice. The obese mice also burnt less energy than their healthy counterparts10. These organelles might help macrophages to function when their metabolism is disrupted, says Brestoff.
In the labyrinthine world of the immune system, donated mitochondria might have an anti-inflammatory effect, especially when they are taken up by T cells — white blood cells that stave off infections and disease. In studies in cell cultures, Patricia Alejandra Luz-Crawford, an immunologist at the University of the Andes in Santiago, Chile, and her colleagues found that some types of T cell that receive mitochondria from stem cells produce fewer inflammatory molecules. Stem cells cultured from people with rheumatoid arthritis pass fewer mitochondria along to T cells than do stem cells from healthy individuals, which she says might contribute to the chronic inflammation associated with the disease11.
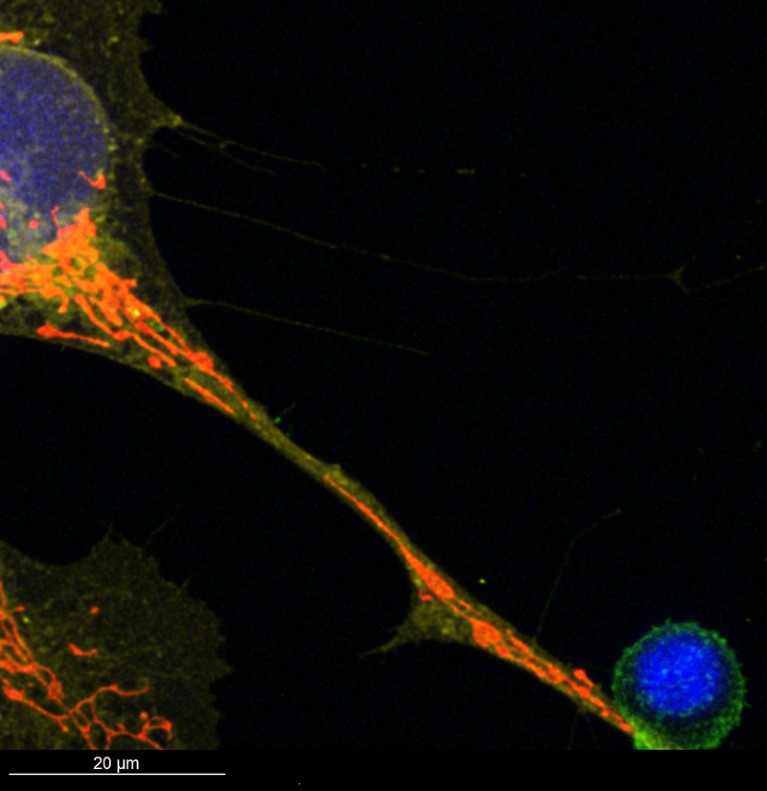
Mitochondria, visible in red, travel through a tunnelling nanotube from a type of bone marrow cell (top left) to a T cell that fights infections and cancer (bottom right).Credit: Jeremy Baldwin
But there are many unanswered questions about mitochondrial transfer, including what the organelles might be doing after they enter cells and how long they last, says Luz-Crawford. “There’s still a lot of mystery.”
The lack of detail about why cells transfer their mitochondria makes it hard to know what specific role these cellular exchanges might have in conditions such as cardiovascular disease and obesity, says Rodriguez. In vivo studies have tracked mitochondria in only a handful of tissue types, making it difficult to build a bigger picture of the wider impacts these transfers have on health.